【溫馨提示】壓縮包內(nèi)含CAD圖有下方大圖片預(yù)覽,下拉即可直觀呈現(xiàn)眼前查看、盡收眼底縱觀。打包內(nèi)容里dwg后綴的文件為CAD圖,可編輯,無(wú)水印,高清圖,壓縮包內(nèi)文檔可直接點(diǎn)開(kāi)預(yù)覽,需要原稿請(qǐng)自助充值下載,所見(jiàn)才能所得,請(qǐng)見(jiàn)壓縮包內(nèi)的文件及下方預(yù)覽,請(qǐng)細(xì)心查看有疑問(wèn)可以咨詢QQ:11970985或197216396
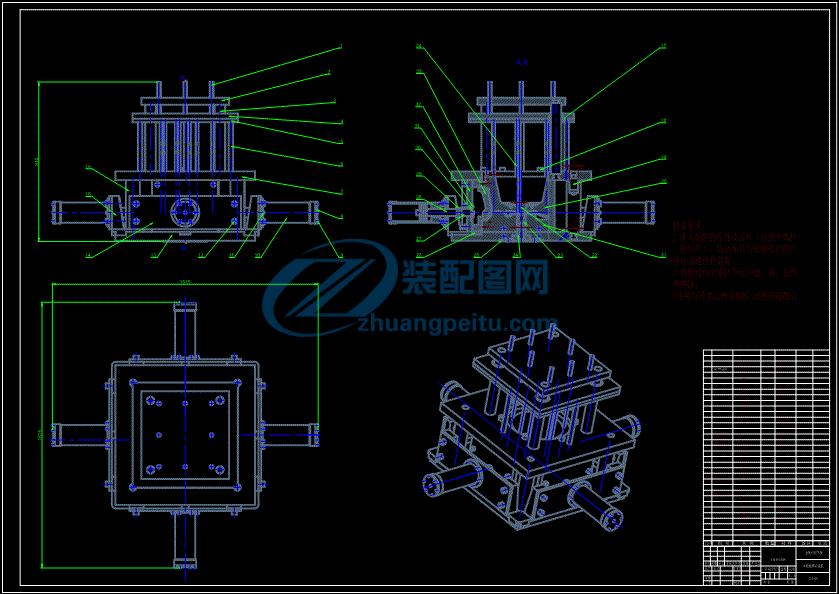
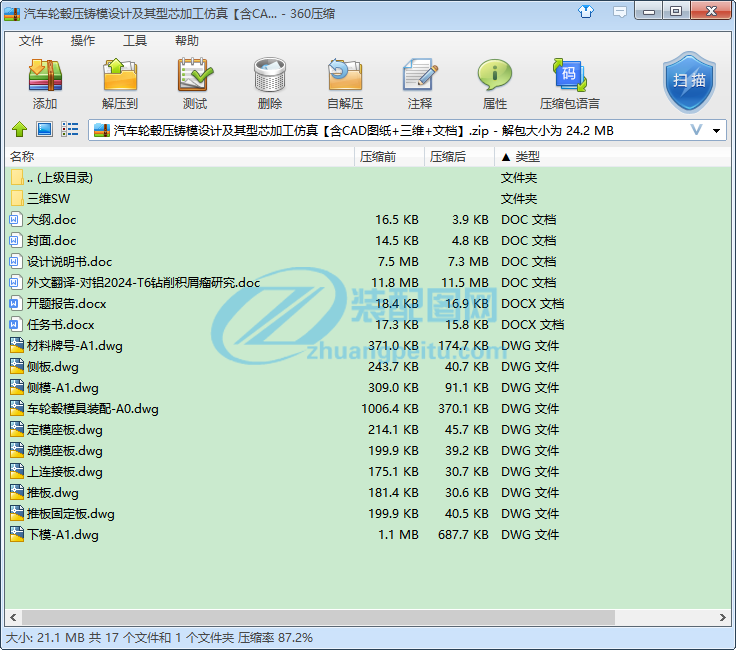
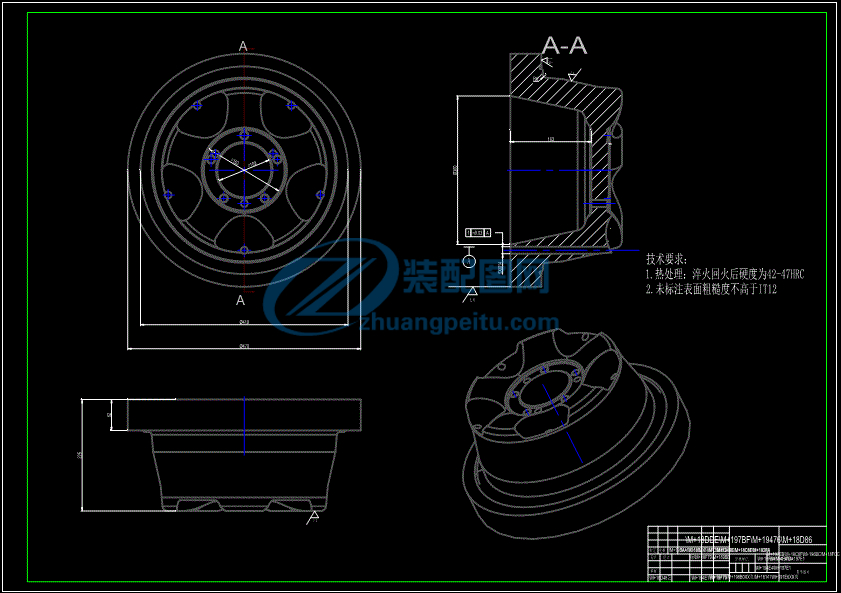
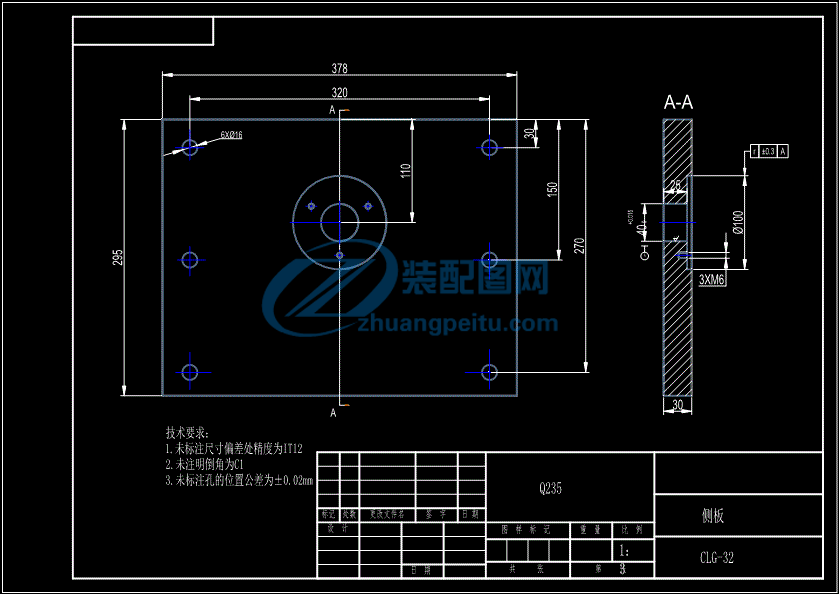
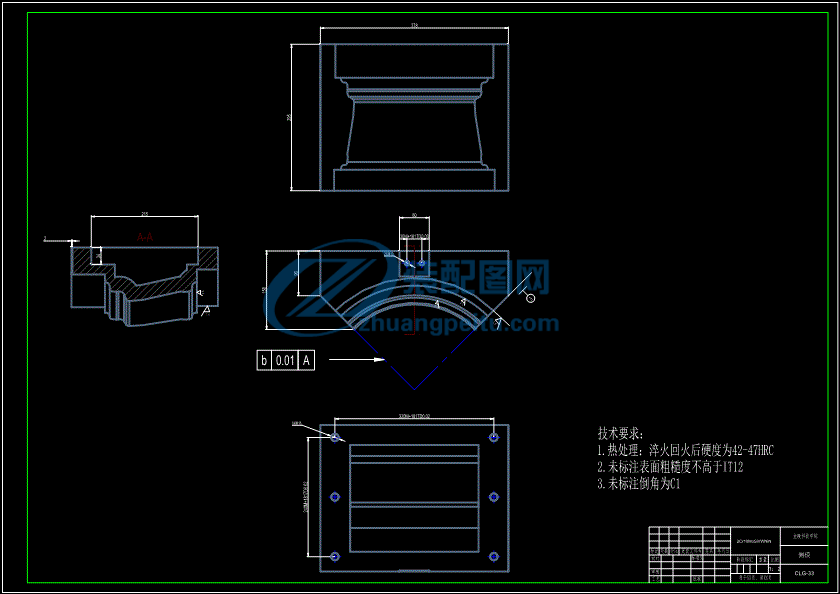
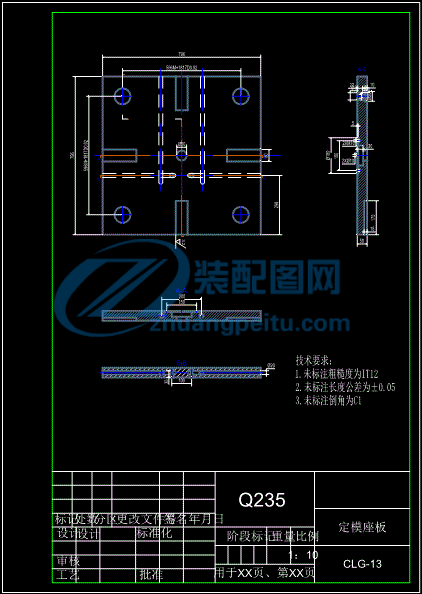
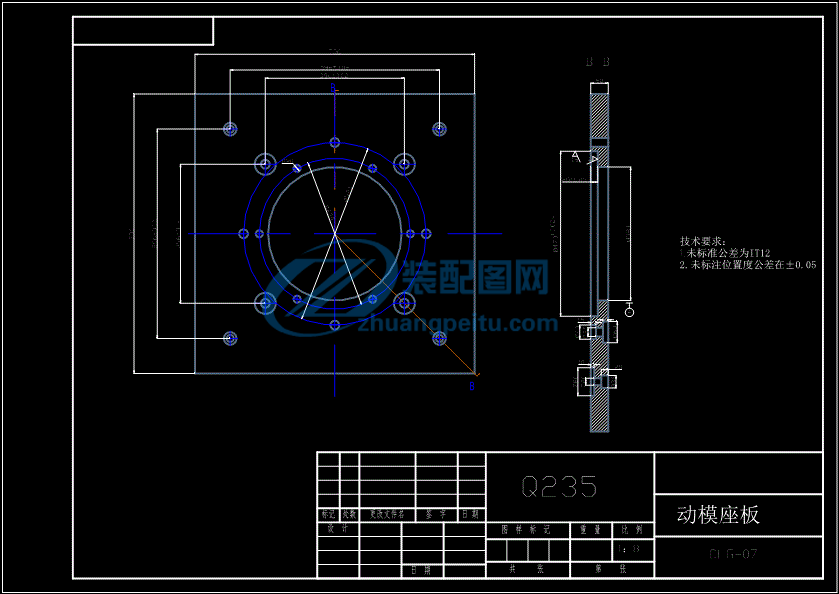
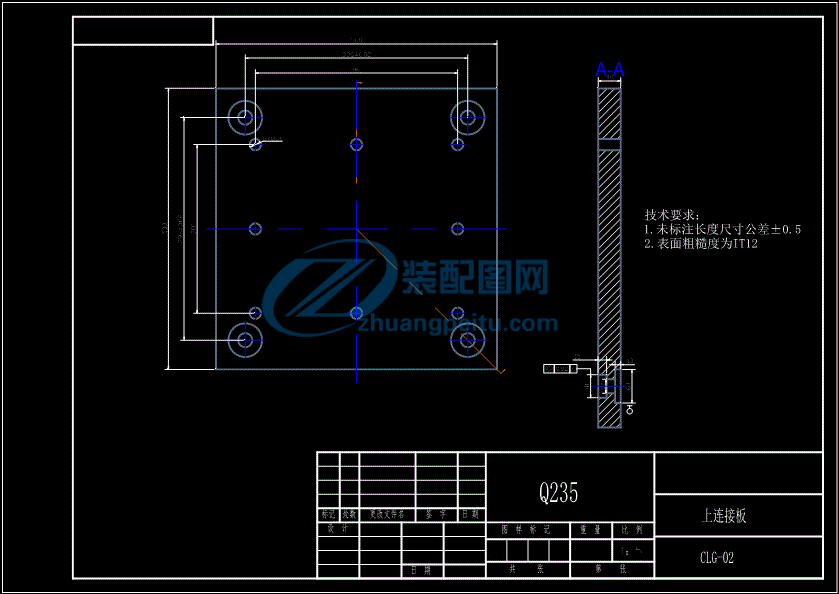
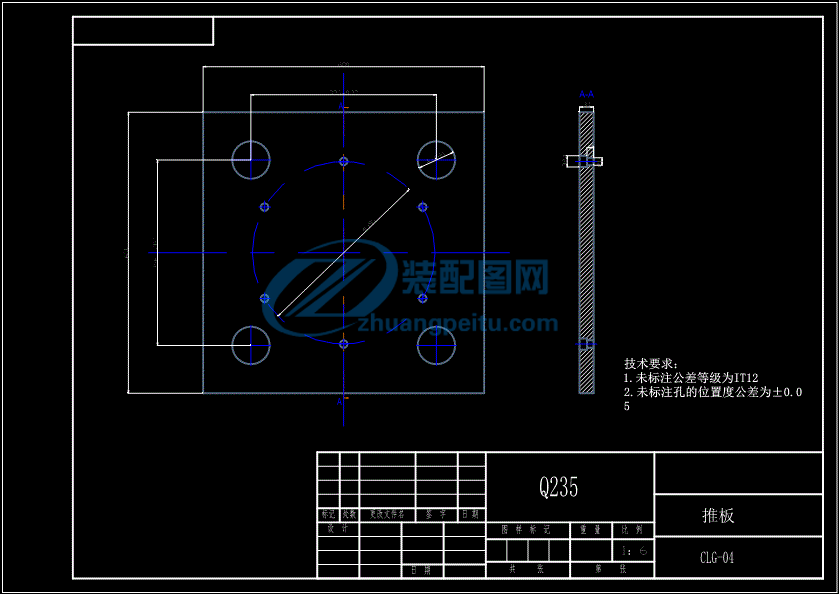
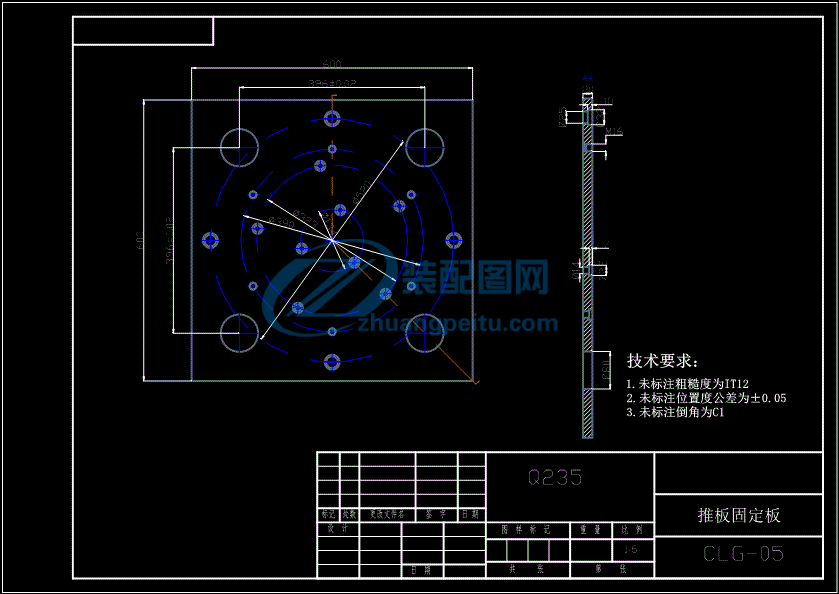
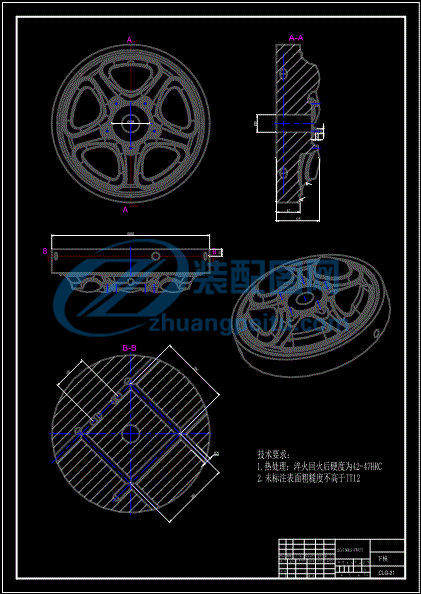
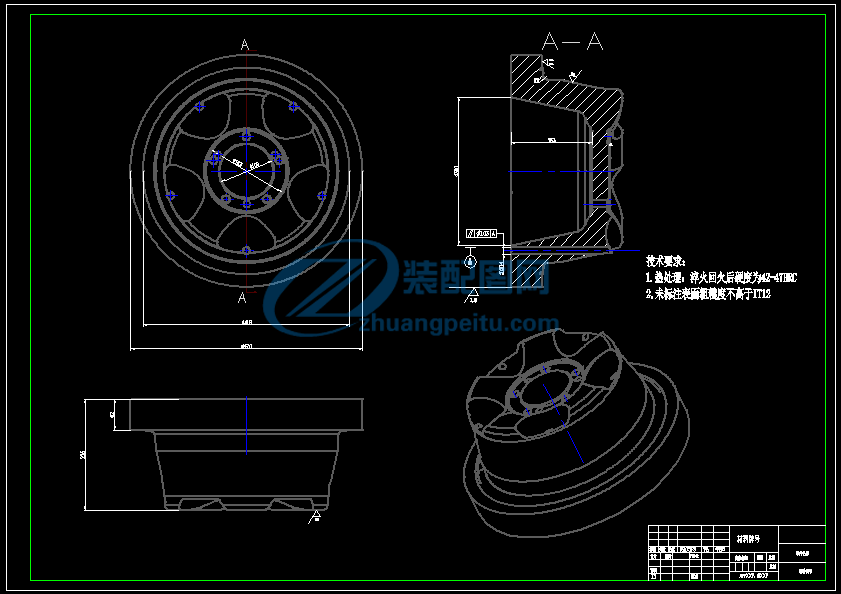
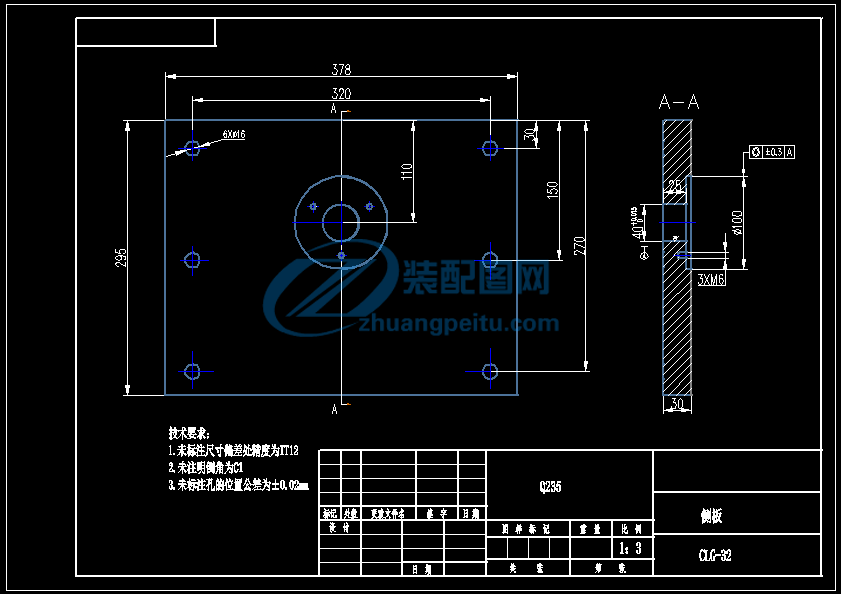
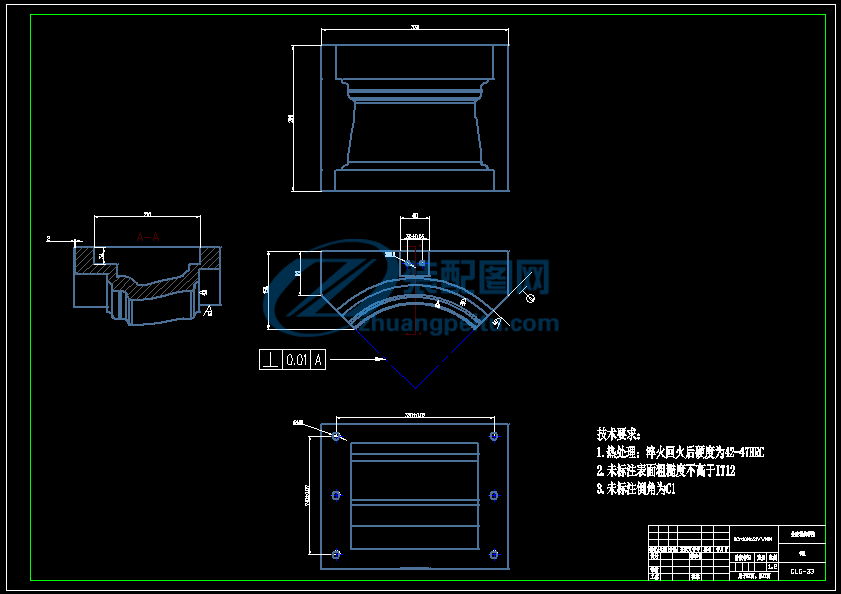
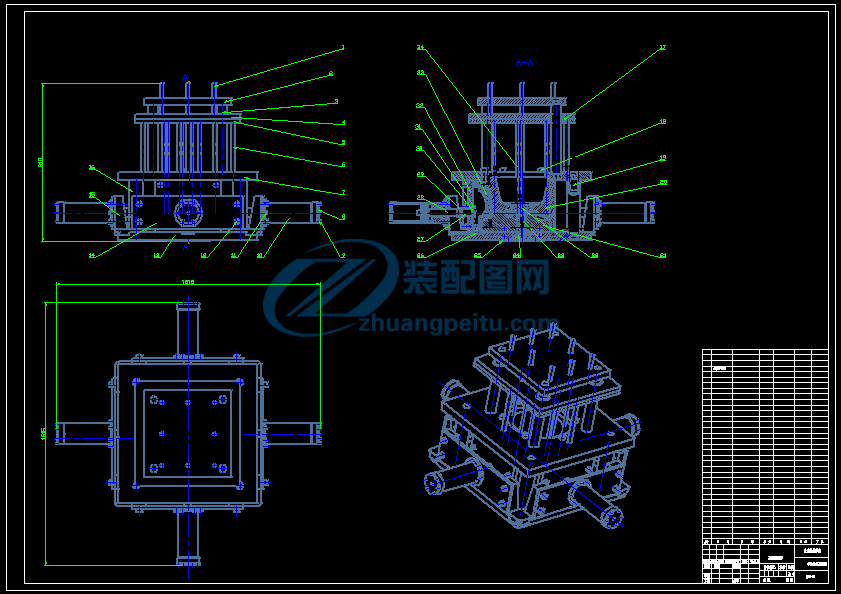

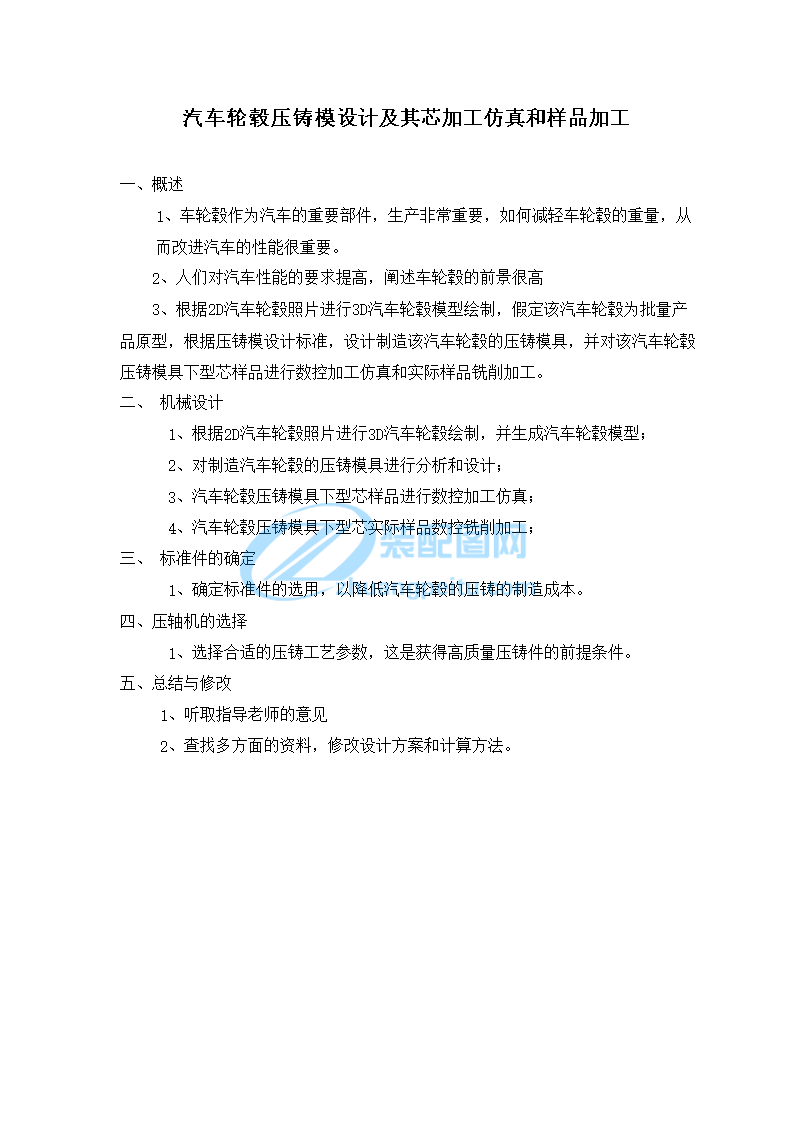

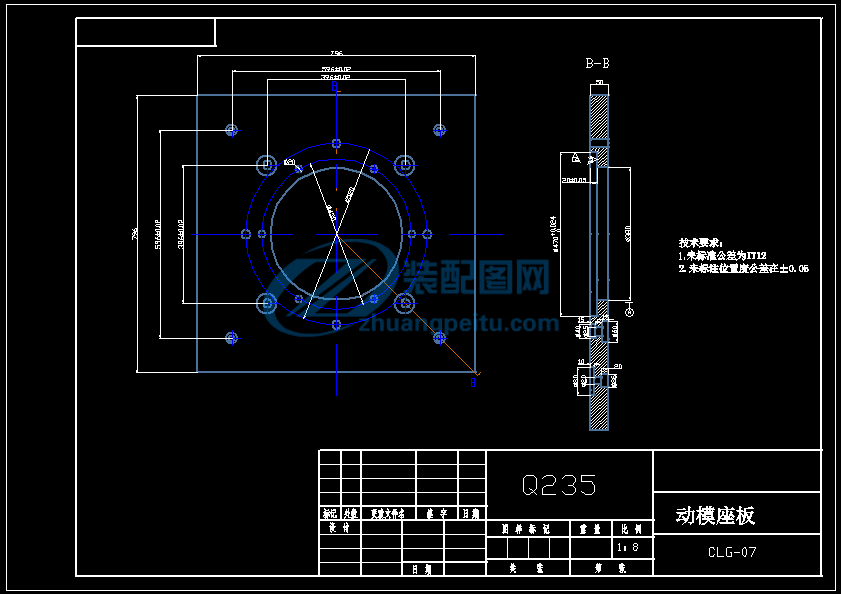
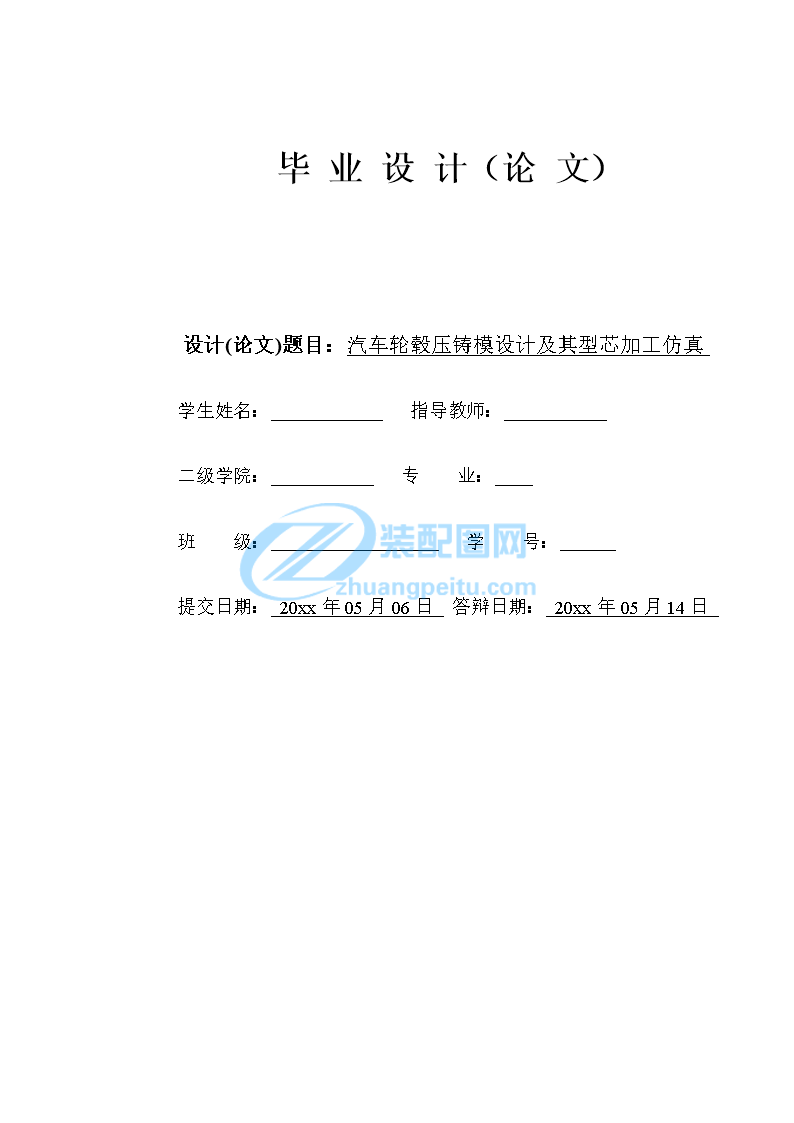
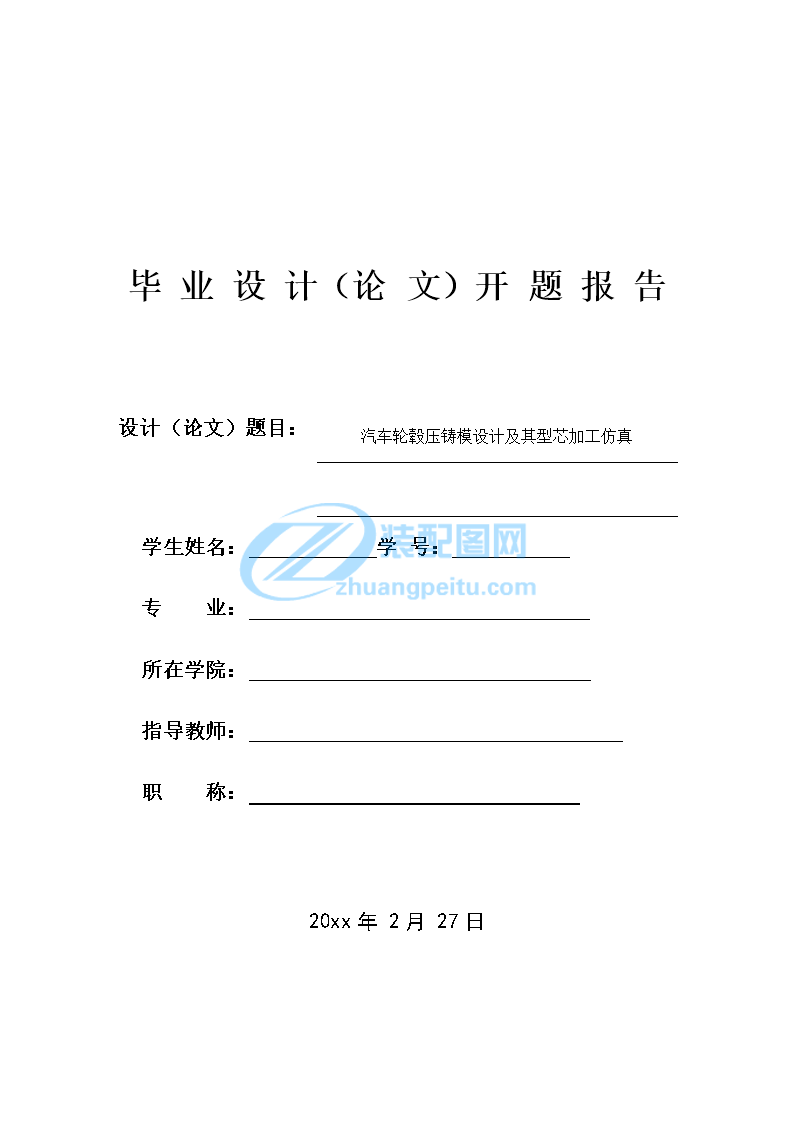
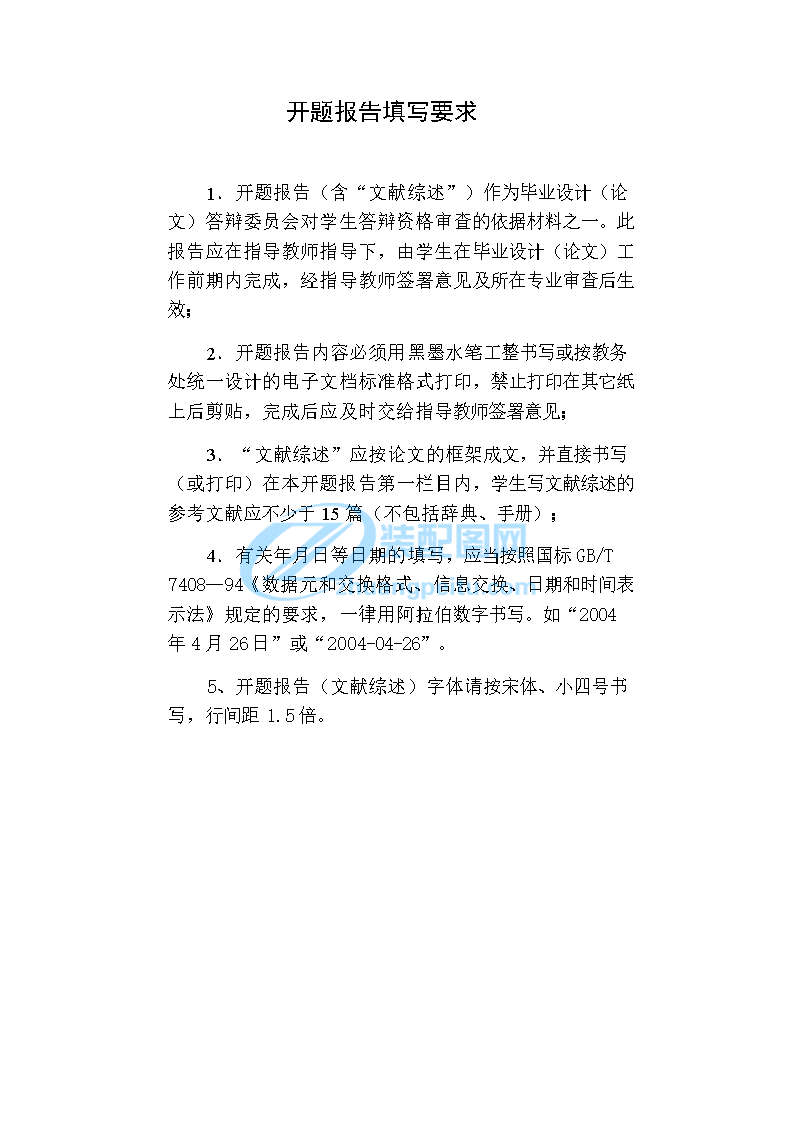
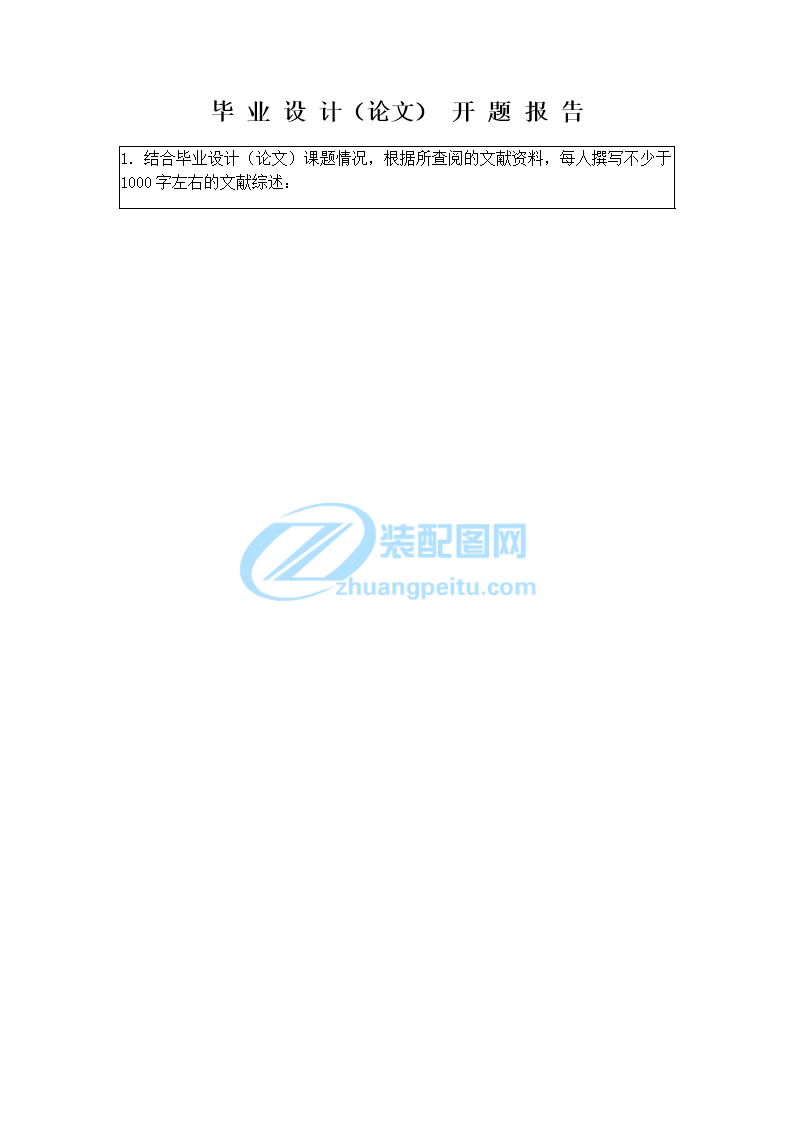
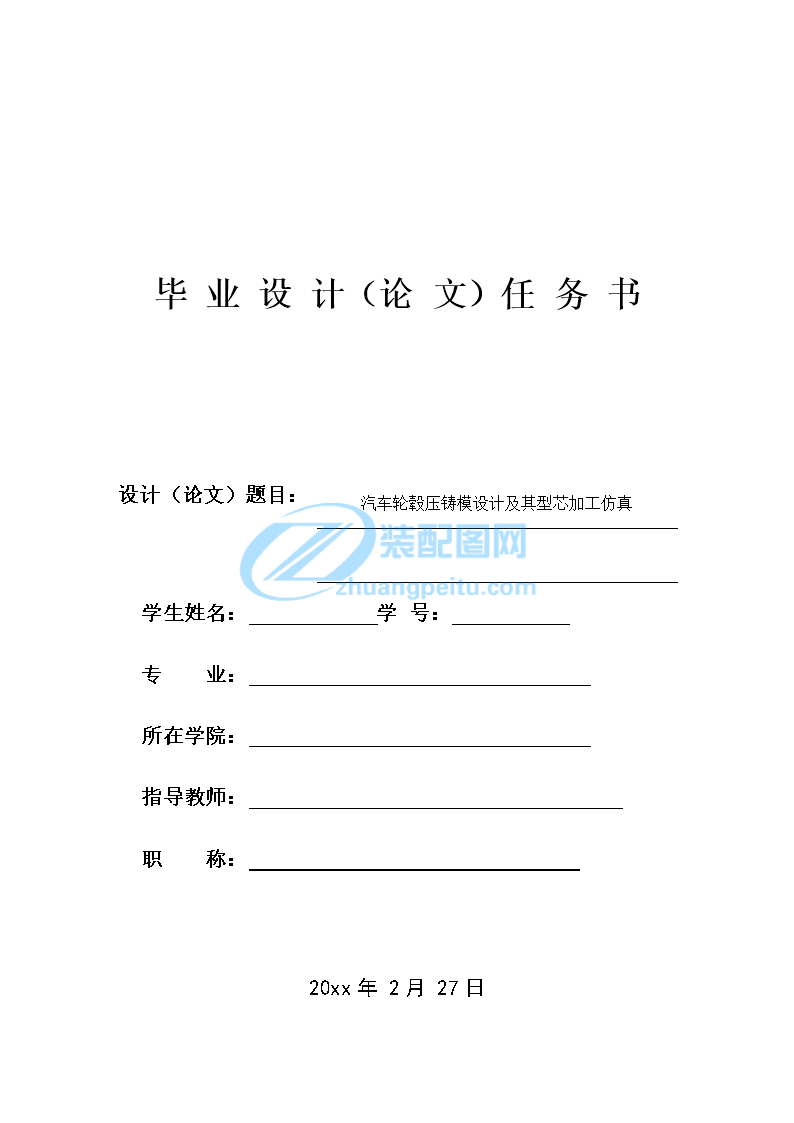
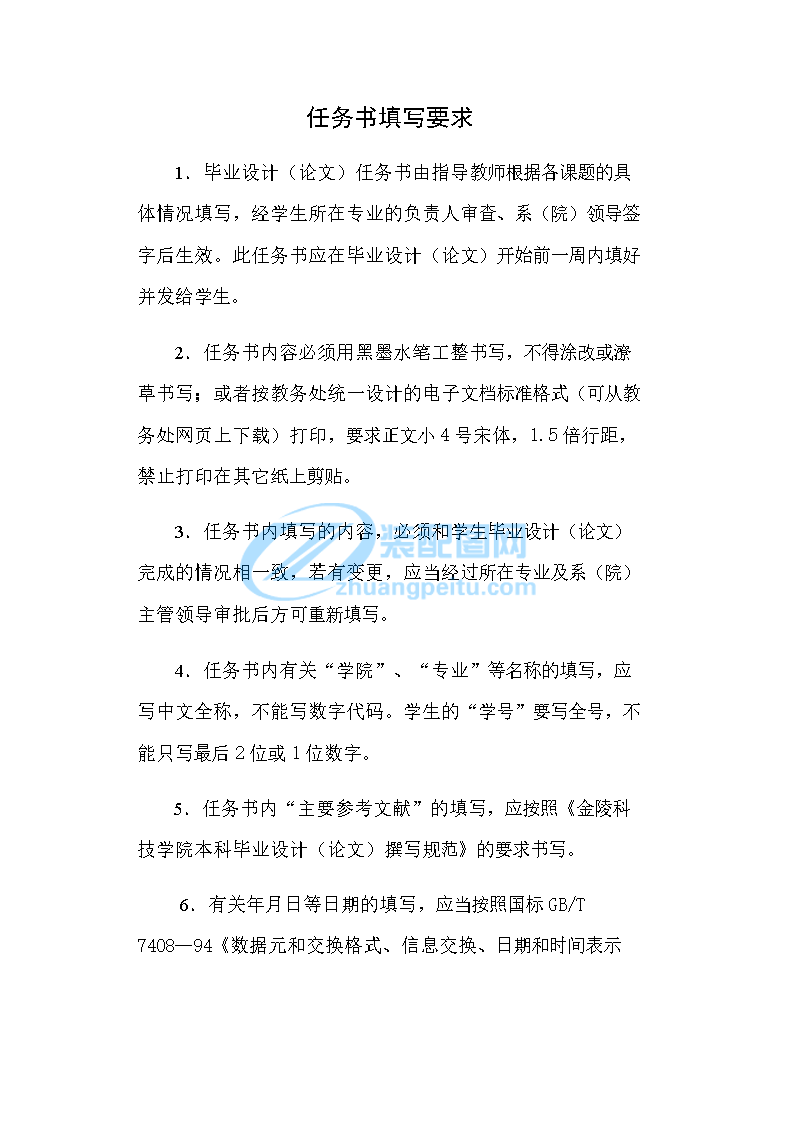
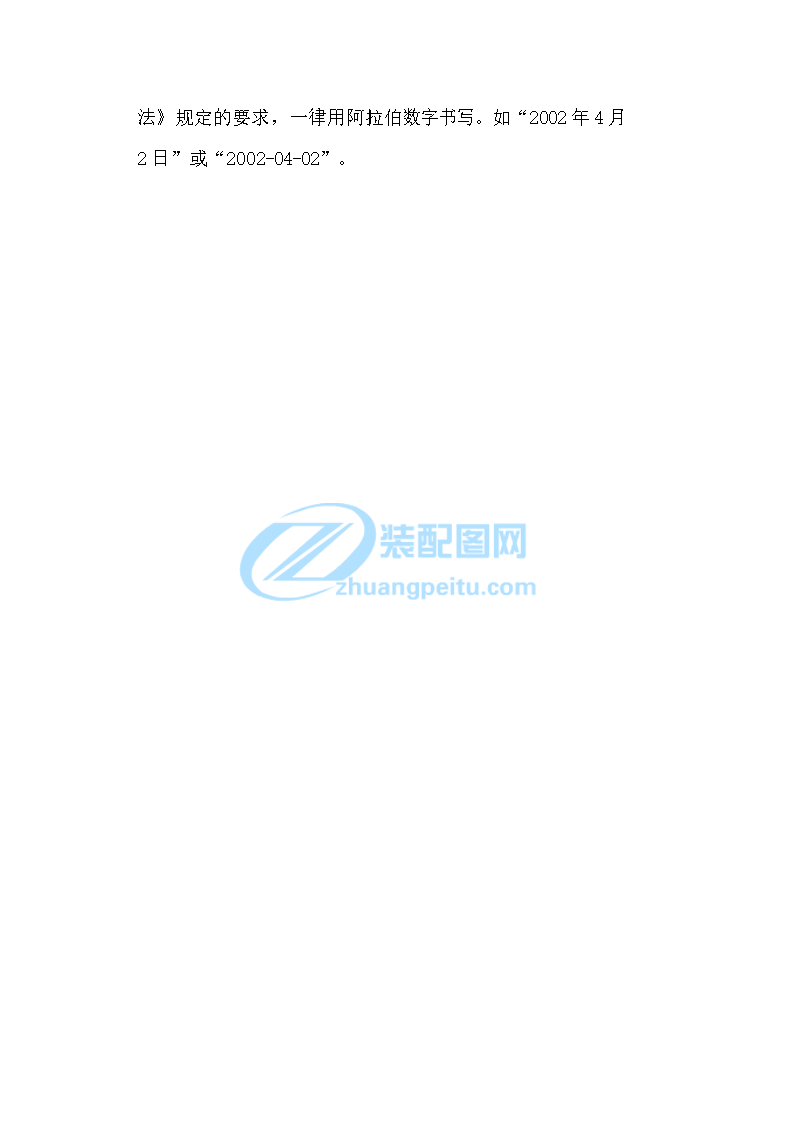
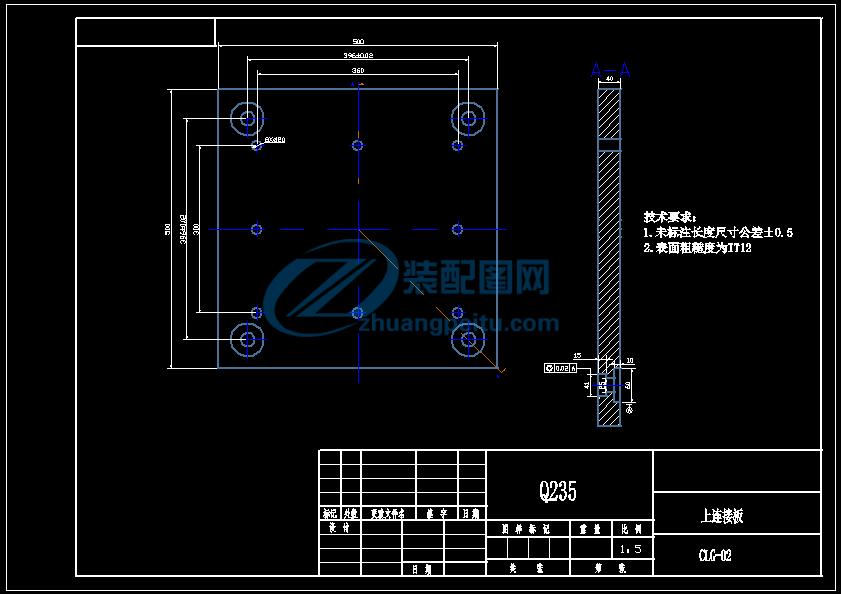
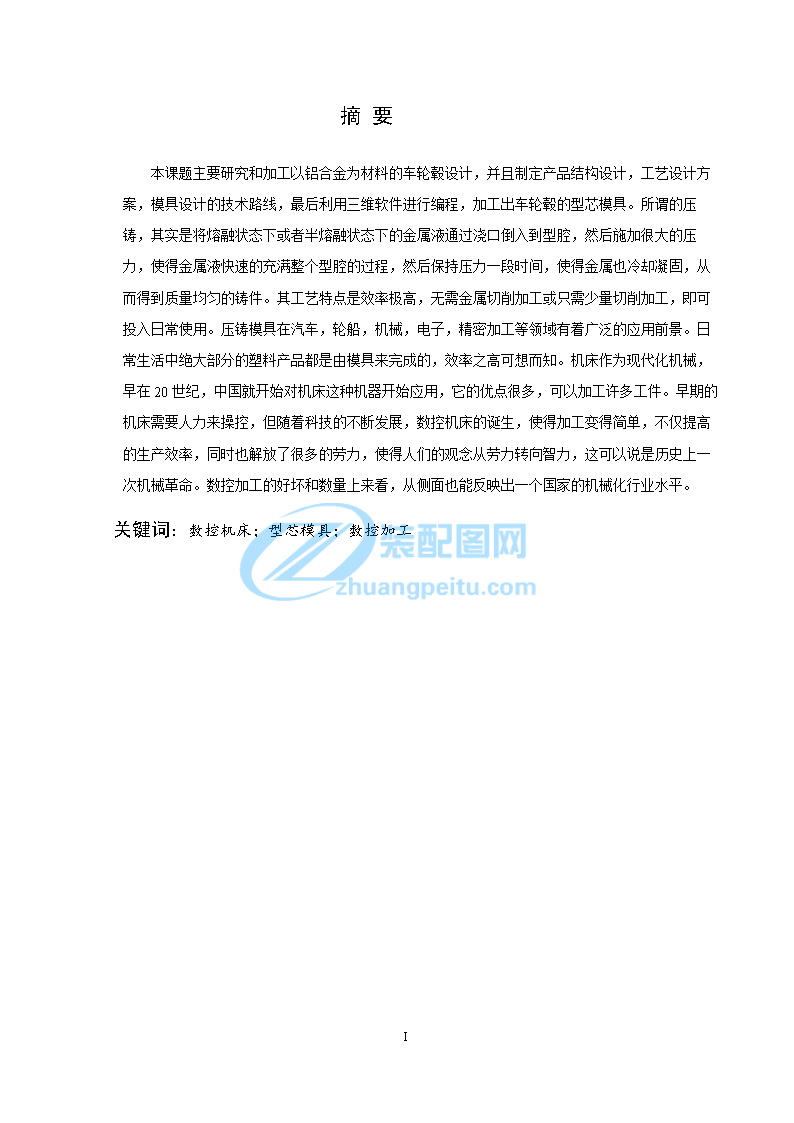
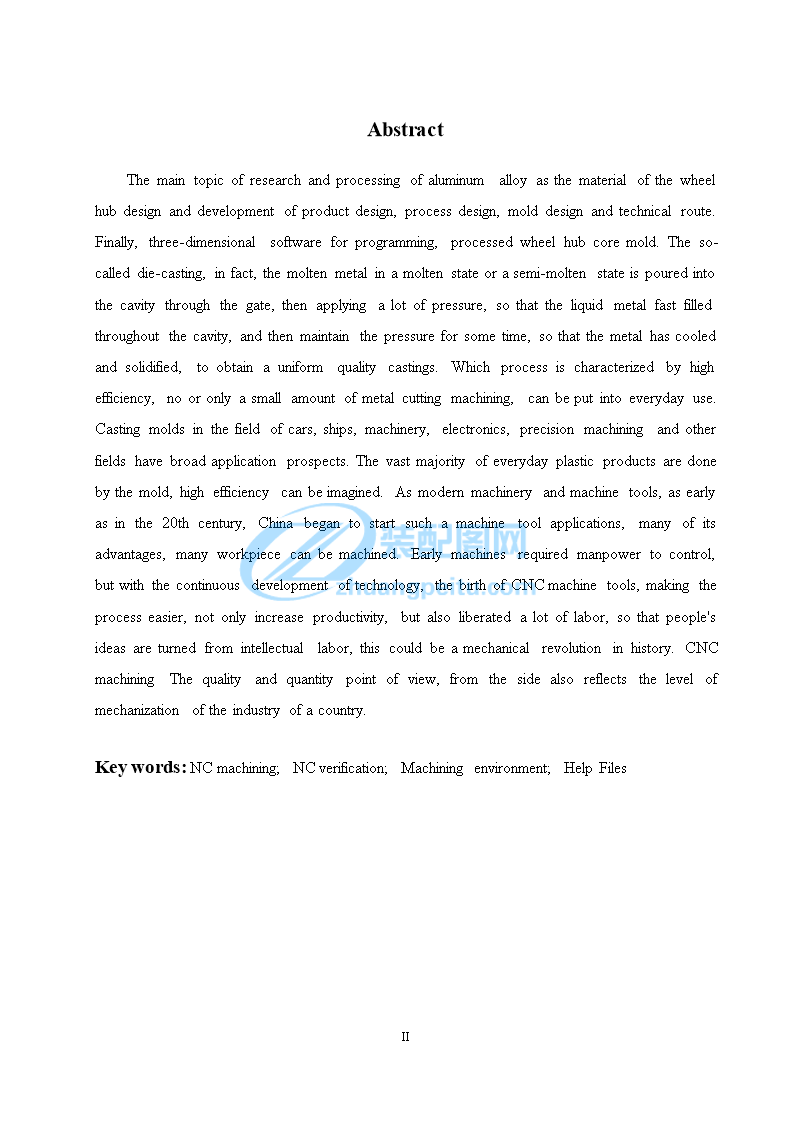
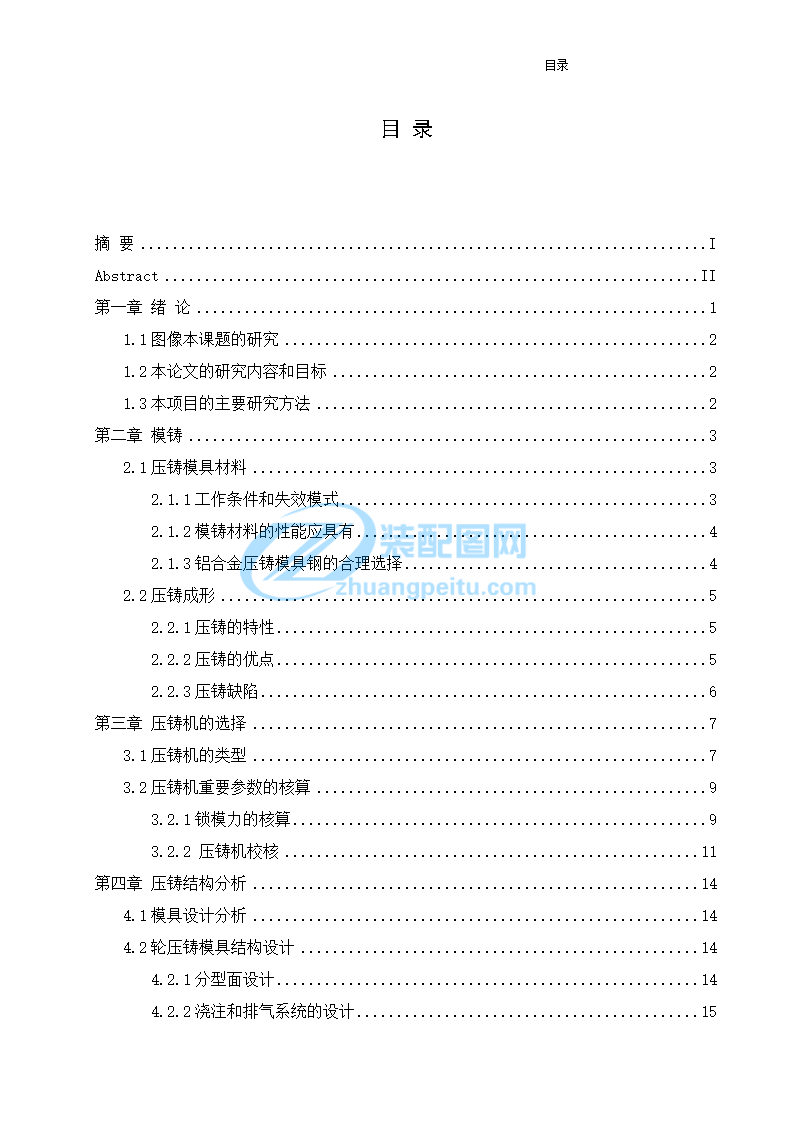
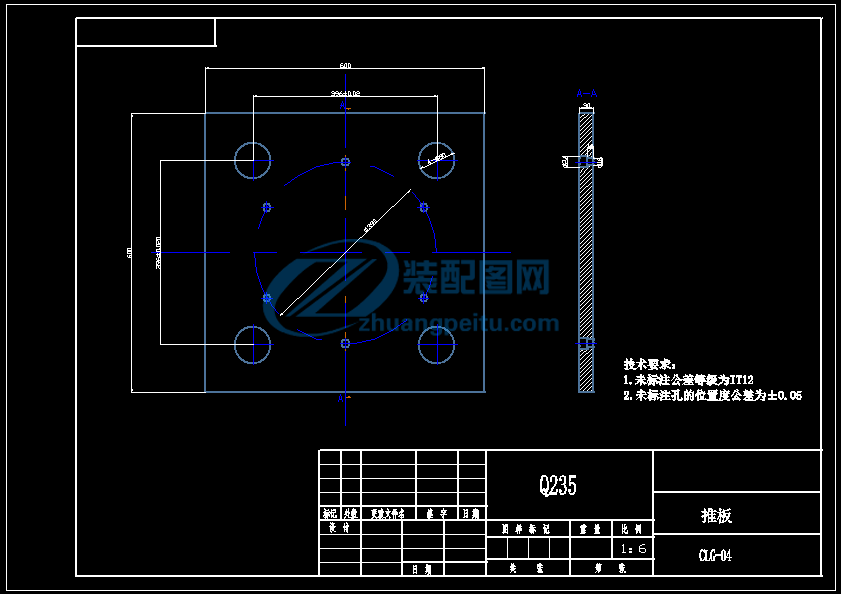
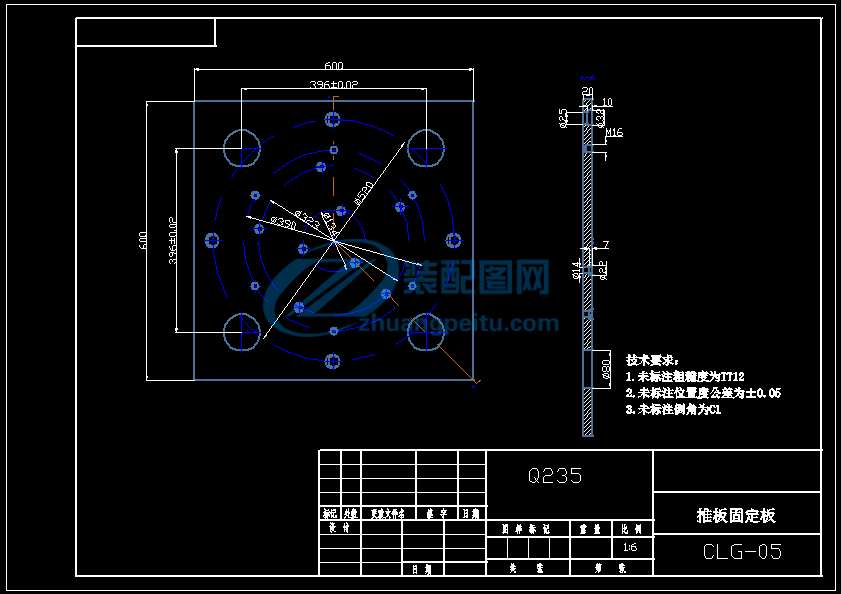
畢 業(yè) 設(shè) 計(jì)(論 文)外 文 參 考 資 料 及 譯 文
譯文題目: Built-up Edge Investigation Invibration
Drilling of Al2024-t6
對(duì)鋁2024-T6鉆削積屑瘤研究
學(xué)生姓名: 學(xué) 號(hào):
專(zhuān) 業(yè):
所在學(xué)院:
指導(dǎo)教師:
職 稱:
20xx年 2月 27日
Built-up Edge Investigation in Vibration Drilling of Al2024-T6
abstract
Adding ultrasonic vibrations to drilling process results in an advanced hybrid machining process, entitled ‘‘vibration drilling’’. This study presents the design and fabrication of a vibration drilling tool by which both rotary and vibrating motions are applied to drill simultaneously. High frequency and low amplitude vibrations were generated by an ultrasonic transducer with frequency of 19.65 kHz. Ultrasonic transducer was controlled by a MPI ultrasonic generator with 3 kW power. The drilling tool and workpiece material were HSS two-?ute twist drill and Al2024-T6, respectively. The aim of this study was investigating on the effect of ultrasonic vibrations on built-up edge, surface quality, chip morphology and wear mechanisms of drill edges. Therefore, these factors were studied in both vibration and ordinary drilling. Based on the achieved results, vibration drilling offers less built-up edge and better surface quality com- pared to ordinary drilling.
Keywords: Vibration drilling、Drilling、Ultrasonic、Aluminum 2024-T6
1. Introduction
Drilling processes constitute a big part of all machining processes (about 40%) [1], which may have a great effect on production costs. A strategy that can reduce these costs is optimizing these processes. A recent method used to improve twist drilling process is adding ultrasonic vibrations to the process. In this method, ultrasonic vibrations are used to improve Ordinary Drilling (OD). This kind of drilling process, that is a hybrid process, is entitled Vibration Drilling (VD) in this paper. In VD the high frequency and low amplitude vibrations are added to OD in direction of feed. Hence, the drill bit has an additional ultrasonic reciprocating motion in the longitudinal direction. Different investigators have reported a variety of improvements in using VD. Babitsky et al. [2] showed that it is possible to drill through thin, ?exible plates with little deformation by VD in contrast to OD where a back support needed to hold plates against thrust force. They explained that the reduction of deformation of plates in VD is because of a discon- tinuous cutting phenomenon which decreases thrust force. Pujana et al. [3] reported that in VD of Ti6Al4 V, chips intend to break. Azarhoushang and Akbari [4] studied the drilling of Inconel 738- LC. They found that the surface quality improves in VD when compared to OD. Other researchers who applied ultrasonic vibrations to machining processes declared that thrust force and torque decreased by these processes [5–9]. Li etal. [10] developed multi-stage control for vibration condition of VD in order to optimize drilling of laminated composite materials. They changed the vibration condition according to the workpiece material being cut and the cutting situation (entrance, middle and exit of the hole). By this technique, the average de?ection of drill point, error of the hole diameter and the exit burr height were reduced by over 20%, 80% and 40%, respectively, in contrast to OD. Liao et al. [11] investigated the VD of Inconel 718. The reduction of chips’ length and torque variations in VD were reported in comparison to OD. Zhang et al. [12] showed that in micro VD in compared with micro OD, tool life increases. They also demonstrated that in VD, because of the drill skidding reduction, hole dimensional accuracy can be improved. Chen et al. [13] developed a voltage controller to keep resonance condition during VD. The reported results showed that by this technique tool life in VD of Inconel 718 enhanced when compared to OD. However, against all mentioned bene?ts for VD, there are some unexpected results. For example, reduction of tool life has been reported in some researches [14,15]. The present researchers believe that much more studies need to be done to know VD. To do this, some drilling experiments performed on Al2024-T6. Use of aluminum alloys in industry is growing because they are lightweight metallic materials and have different interesting mechanical and thermal properties [16]. But, in dry machining of these alloys, the major problems are built-up edge formation and low quality of generated surface [16–20]. These problems are more critical in producing of precision workpieces. In this work, built-up edge in VD of Al2024-T6 is studied in detail. Also, the effect of VD on surface quality is investigated and compared to OD. The in?uence of machining condition and built-up edge on chip morphology is also presented.
2. Vibration drilling
Fig. 1 shows a schematic con?guration of 1D vibration drilling.
According to this ?gure, tool vibrates in drilling operation longitudinally. To impose ultrasonic vibration motions on drill bit, there are three different systems: (1) A system in which drill vibrates and workpiece rotates [4]. In this system the rotary motion is applied on workpiece by lathe jaw chuck and the vibrating set (transducer, booster, horn and drill) is ?xed on the support of lathe machine. By using this system, drilling of workpeices that can be clamped by jaw chuck, would be possible. (2) A system with vibrating workpiece and rotating drill [21]. In this system, vibrations are induced to workpiece, therefore, for each new workpiece (with different geometry and material) to be cut a new modal analysis and design are necessary. Hence, the system is only applicable to experimental purposes. (3) A system in which both vibration and rotation are applied to drill [3]. In this system, vibration tool rotates and workpiece is fed toward the tool. Drilling various workpieces with different materials, geometry or dimensions is possible by this mechanism. This system is also more capable to be industrialized. In this study, the third system was selected to perform VD.
3. Experimental set-up
In vibration machining processes, designing a vibrating tool which induces the resonance frequency is required. The resonance frequencies of any parts can be found by modal analysis. To achieve longitudinal resonance frequency in VD the modal analysis was performed on the model of vibration tool (horn and drill). As the drill geometry is asymmetric and the results of a 3D analysis can be much more accurate, the 3D models of vibration tool were prepared in CATIA software, and then, transferred to ABAQUS software to do modal analysis. Since geometries of drills were standard and HSS was chosen as tool material because this material has shown good results in drilling of aluminum alloys as [14,22–24] have reported, tool geometry and material were ?xed parameters. Hence, the alternative to vary designs in order to change longitudinal resonance frequency of vibration tool and matching it to the longitudinal frequency of transducer-booster set was horn geometry. This geometry in software was changed as long as the vibration tool longitudinal frequency
Fig. 1. Model of VD.
came near to 19.65 kHz that is the longitudinal resonance frequency of transducer-booster set.
Table 1 shows the tool and horn material speci?cations.
Vibration tool model was meshed by TETRA elements (Fig. 2).The smaller the mesh size, the more accurate results can be achieved, therefore, the mesh size changed as far as the error between the two ?nal consecutive analyses turned into 2%. The next step after meshing is performing modal analysis. The modal analyzer of the software was used and modal analysis was performed.
Fig. 3 shows the longitudinal mode shape of the designed vibration tool. The resonance frequency of the longitudinal mode is 19.665 kHz which is close to the longitudinal resonance frequency of transducer-booster set.
After the ?nal design, a vibration tool, considering the achieved dimensions from analyses was fabricated and connected to the transducer-booster set (Figs. 4 and 5).
Since the ultrasonic generator, which was used during the experiments, was able to scan the resonance frequencies, the vibration tool set was scanned to ?nd its longitudinal resonance frequency in practice. Fig. 6 shows the charts achieved by the scan of transducer-booster set, and vibration tool set. It can be seen that the frequency of vibration tool set is 19.99 kHz. The negligible difference between the frequency of transducer-booster set and vibration tool set is due to errors in material properties, manufacturing and assembling of tool.
In the next step, a rotary mechanism with the ability to induce both rotation and vibrations to drill was designed. In this mechanism, vibrating set was placed in a steel tube and the tube clamped by jaw chuck. The electricity was transmitted to transducer via two brushes that were in connection with two copperrings and were located in
Fig. 2. Meshing the vibration tool.
Fig. 3. Mode shape of longitudinal resonance frequency.
Fig. 4. A schematic picture of vibration tool set.
Fig. 5. A picture of vibration tool set.
Fig. 6. Scan charts gained by ultrasonic generator: (a) Scan chart of transducer-booster set.
(b) Scan chart of vibration tool set.
the back of the lathe machine. The workpiece was clamped through a ?xture on the lathe machine support. Fig. 7 shows the VD set-up.
Experimental equipment is as follows:
? Universal lathe machine (AJAX-AJ 725): to perform drilling experiments.
? Ultrasonic generator (MPI WG-3000 W): to convert low frequency electrical current to high-frequency electrical current.
? Laser vibrometer (OMETRON-VH3000+): to measure vibration amplitude.
? Dynamometer (KISTLER-9257B): to measure thrust force.
? Optical microscope (Dino-Lite Digital Microscope): to investigate the built-up edge on tool surface.
? Roughness tester (Mahr, Perthometer M2): to measure surface roughness.
? Drill: diameter of 5 mm made of HSS.
? Workpiece material: aluminum 2024-T6 (20 × 30 × 85 mm3).
Fig. 7. VD set-up.
Fig. 8. Experimental equipment. (a) Lathe machine (AJAX-AJ 725). (b) Ultrasonic generator
(MPI WG-3000 W). (c) Laser Vibrometer (OMETRONVH3000+). (d) Dynamometer (KISTLER-9257B). (e) Optical microscope (Dino-Lite Digital Microscope). (f) Roughness tester (Mahr). (g) HSS drills. (h) Workpeice.
Fig. 9. Thrust force vs. hole number (460 RPM, a = 0). Fig. 10. Thrust force vs. hole number (460 RPM, a = 10 lm).
4. Experiments and results
Some drilling experiments were performed on Al2024-T6 workpieces and built-up edge, chip morphology and surface quality were studied and compared between OD and VD. All experiments were done without lubricant (i.e. dry cutting).
To investigate the in?uence of machining parameters, three rotary speeds and three feed rates were chosen for two processes. The values of these parameters are represented in Table 2. Zero amplitude in this table indicates OD conditions. With a new drill, 10 holes with 20 mm depth of cut were made on workpiece for each machining condition. Since built-up edge in?uences thrust force, it was studied by comparing thrust force increase between two processes and the built-up edge size was also measured by an optical microscope.
Fig. 23. Chip morphology vs. feed rate: (a) 755 RPM, 0.104 mm/rev, a = 0. (b) 755 RPM, 0.348 mm/rev, a = 0.
Fig. 24. Surface roughness vs. feed rate.
4.1. Results
Figs. 9–14 show thrust force diagrams in different machining conditions. Each chart plots thrust forces for constant rotary speed and amplitude, and three different feed rates. In these charts horizontal axes represent number of drilled holes and vertical axes present thrust forces. On the other words, number 1 is related to the ?rst hole made by a new drill and numbers 2–10 are the next holes produced by the same drill. It can be seen that thrust force increases for higher feed rates. In general cases, by increasing of feed rate, uncut chip thickness increases and results in increasing of chip thickness and cutting forces. Therefore friction between chip and rake face increases and consequently aluminum adhesion to drill cutters happens.
Thrust forces at constant feed rate and different rotary speeds are observed in Figs. 15–20. By increasing the number of holes, thrust force increases which is due to adhesion presence enhancement and built-up edge formation.
Figs. 21 and 22 show the difference between the amount of forces in the ?rst hole and the tenth one (force increase value). According to these ?gures, force increase value increases as the feed rate increases. The reason is aluminum adhesion on drill cutting edges and built-up edge presence in cutting zone; resulting in friction increase and rake angle decrease (tool blunting happens). Therefore the more feed rate, the more adhesion of work material to the tool. Another observation is that the force increase value is less in VD when compared to OD; that is due to the friction reduction in VD which can reduce the formation of built-up edge.
In Figs. 21 and 22, it can be seen that by increasing rotary speed the force increase value decreases that is a result of uncut chip thickness reduction which happens by increasing of rotary speed, and then, results in machining forces decrease. Therefore, the bigger rotary speed, the smaller force increase value and the less builtup edge.
Figs. 21 and 22 also illustrate the less increase of force increase value in VD in contrast to OD. This result is because of adhesion reduction by adding ultrasonic vibration to the process (VD). Also, as the adhesion and built-up edge presence decrease, a reduction
Fig. 25. Chip flocking to drill flutes. (a) 460 RPM, 0.208 mm/rev, a = 0. (b) 460 RPM, 0.208 mm/rev, a = 10 lm.
Fig. 26. Holes surfaces. (a) 755 RPM, 0.208 mm/rev, a = 0. (b) 755 RPM, 0.208 mm/rev, a = 10 lm.
in force increase value happens as a function of rotary speed increase.
The effect of feed rate on chip morphology is shown in Fig. 23. Chips breakage happens as feed rate increases. It should be noted that although shorter chips have smaller contact with tool, however, since uncut chip thickness increases as feed rate increases, the machining forces are become larger and the chips move on rake face much more roughly which results in more adhesion, and consequently, higher force increase values.
In the present work, the in?uence of VD on surface roughness is investigated as well. A comparison has been made among roughness of the ?rst holes of every 10-hole set. The results are shown in Fig 24. It can be observed that by increasing of the feed rate the surface roughness becomes worse. However, as the rotary speed increases, better surface qualities can be obtained. The reason of obtaining worse surface quality as feed rate increases is higher forces which increase drill skidding and instabilities in drilling process. But, because of smaller uncut chip thickness and lower machining forces, the stability of drilling process increases by enhancing rotary speed and leads to less drill skidding. However, drill skidding makes chips movements irregular and causes chips to strike and scratch holes surfaces.
It is also obvious in Fig. 24 that holes produced by VD have better surface ?nish. This improvement is because of friction reduction between hole’s surface and drill shank. Astashev and Babitsky in [25] demonstrated the effect of vibrations on dry friction behavior of a contact surface between a bar and a carriage moving on it. They have shown that whenever vibrations induce (in the direction of feed) between two bodies’ contact surfaces in a system, the system behavior in respect to static force in vibrating conditions is similar to its motion in a linear viscous medium. This phenomenon is recognized as effect of vibrational smoothing of nonlinearities. It may be concluded that the contact between hole’s surface and drill shank is affected by the effect of vibrational smoothing of nonlinearities, therefore, VD should produce better Ra values compared to OD. Furthermore, this phenomenon (the ef-fect of vibrational smoothing of nonlinearities) happens on the contact surface between surfaces of chips and drill ?utes. As the axial component of friction force between the surfaces of chips and drill ?utes decreases, causes chips to move out of drilling hole much easier. In addition, since chips are shorter in VD, because of ultrasonic impact phenomenon, the chips move in drill ?utes much easier and the impacts between chips and hole surface decrease. Therefore, it might result in less adhesion of chips on holes surfaces.
Fig. 27. Adhesion to hole surface. (a) 755 RPM, 0.104 mm/rev, a = 0. (b) 755 RPM, 0.104 mm/rev, a = 10 lm.
Fig. 28. Optical microscope photos of drills bits.
Fig. 29. Chip surface affected by built-up edge. (a) Chip of first hole, 1255 RPM, 0.348 mm/rev, a = 10 lm.
(b) Chip of tenth hole 1255 RPM, 0.348 mm/rev, a = 10 lm.
Fig. 30. Chip morphology with hole number. (a) First hole, 755 RPM, 0.104 mm/rev, a = 0. (b) Tenth hole, 755 RPM, 0.104 mm/rev, a = 0. (c) First hole, 755 RPM, 0.104 mm/rev,a = 10 lm. (d) Tenth hole, 755 RPM, 0.104 mm/rev, a = 10 lm.
Fig. 31. SEM photos of cutting edges. (a) 460 RPM, 0.104 mm/rev, a = 0. (b) 460 RPM, 0.104 mm/rev, a = 10 lm. (c) 755 RPM, 0.104 mm/rev, a = 0. (d) 755 RPM, 0.104 mm/rev,a = 10 lm.
On the other hand, in OD, chips’ movements in drill ?utes are dif?cult and sometimes causes chip ?ocking to ?utes (Fig. 25(a)) since a higher friction happens between continuous chips and ?utes’ surfaces. In OD, chips also strike with holes’ surfaces strongly and make surface quality worse than that in VD. Fig. 26 shows surfaces of holes produced by OD and VD. According to this ?gure, holes produced by VD have better surfaces. In contrast to Fig. 26(b), there are more grooves on holes’ surfaces in Fig. 26(a). This is because of strong impacts that occur between continuous chips and holes’ surfaces in OD.
Generally, built-up edges in machining processes affect surface roughness and chips morphology; because built-up edge pieces separate from cutting edge during machining, and then, adhere to machined surface. In drilling, since there is no direct contact between cutting edges and holes’ surfaces, built-up edge does not have any effect on surface quality, directly. In drilling, adhesion of chips moving through drill ?utes to holes’ surfaces is a damage factor. Fig. 27 compares the adhesion of chips to holes’ surfaces. The adhesion to holes’ surfaces which is produced by OD is much more considerable when compared to VD. This outstanding difference is because of more contact time between continuous chips and holes’ surfaces in OD.
Fig. 28 shows the photos taken by optical microscope from drills’ bits after producing 10 holes. In these photos, adhesion of aluminum to drills’ bits is obvious. As seen, less built-up edges are formed on tools in VD. The explanation is that friction between tool and chips decreases in VD. In other words, vibrations increase macroscopic viscosity coef?cient of contact surfaces which results in seizure length reduction (seizure zone is a place on rake face that chips cease and adhere to tool while moving on rake face) [26]. This reduction of seizure length affects friction reduction in machining processes signi?cantly and causes a reduction in force and chip adhesion to the tool. In addition, because of VD origin, involvement time between tool and work material in VD is much less than in OD which can lead to adhesion reduction.
Principally, in drilling with twist drills the material in front of drill bit is pushed to sides by chisel edge with the h